Cognitive Load Theory: Why Educators Should Leverage It to Optimize Learning
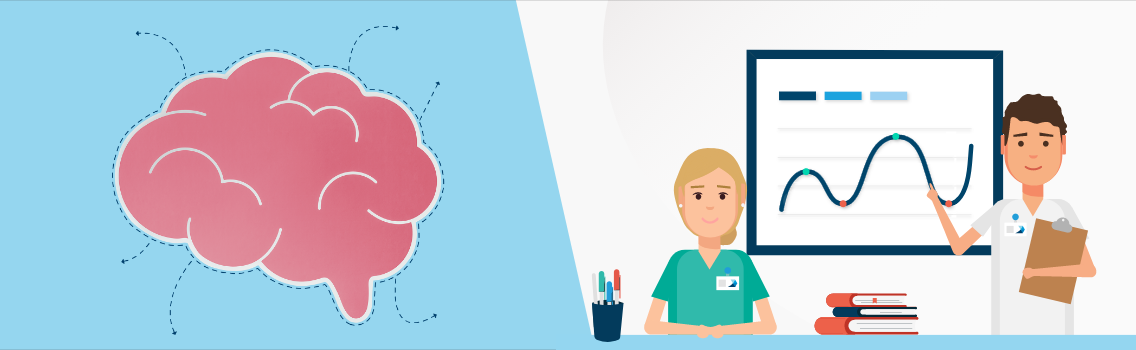
Healthcare providers often have to remember the physical assessment findings of a patient until they can chart, or recall complex medical knowledge to apply in practice when diagnosing and managing a patient.
In these two scenarios, different parts of the brain’s memory functions are called into play: In the former, the information only needs to be retained for a short period of time so utilizing the working (short-term) memory will suffice. The latter requires tapping into long-term memory to retrieve information that has already been learned.1 However, working memory is limited in capacity.2 and in duration3 Long-term memory, on the other hand, provides the ability to store an unlimited amount of information that can be retrieved for later use4—essential for learning and retaining new knowledge—and serves as the foundation that’s critical for constructing higher-order thinking.
Ensuring learners master and retain the information taught for the long term often presents a challenge in healthcare education programs, due to the complexity and rigorous nature of the curriculum. Educators and learners can overcome this and teach and learn more efficiently by understanding how short- and long-term memory work. The key to effectively leveraging both types of memory is to know when and how to employ which. Cognitive load theory has the answers.
Understanding Working and Long-Term Memory
Developed in the 1980s by psychologist John Sweller, cognitive load theory builds upon the concept of human information processing or cognitive architecture to explain how we learn, think, and solve problems.5 It involves both working memory and long-term memory: incoming information is registered in our sensory memory before being channeled to the working memory to be processed for meaning (through reasoning and comprehension). The information is then committed into long-term memory, or discarded.
In short, new information must first be processed by our short-term memory before it can be stored in long-term memory for later use. But why does working memory have such short-lived efficacy whereas long-term memory is limitless?
Let’s use the analogy of a computer’s RAM (random-access memory), the short-term memory storage of the CPU (central processing unit). Downloading new or additional software onto your computer will eat up RAM, causing the system to slow down and become less efficient.
Similarly, our brains require great mental effort and resources to learn new material. The amount of mental activity that the working memory has to perform is known as cognitive load. With its small capacity—it can only remember three to five chunks of information at a time6—working memory gets overwhelmed as the load builds up. Likewise, when learners are presented with too much information, their brain can suffer from overload, thereby slowing down or halting learning altogether.7
For effective and efficient learning, cognitive load theory suggests that instructional methods should focus on extending the working memory.
Building Schema: The Importance of Recalling and Embedding Knowledge
When a learner intakes new information, the brain creates a mental framework to understand and interpret the data by organizing and linking related pieces of knowledge. This cognitive structure, called schema, reduces cognitive load by allowing the mind to draw upon existing knowledge and activate linked memories, concepts, or words. For instance, when healthcare providers encounter familiar elements in treating patients, they can recall related knowledge to facilitate accurate clinical diagnosis.8
The more schemas the learner has in their long-term memory, the better they can link new information to existing knowledge, thus minimizing cognitive load and stretching their working memory to accommodate more learning. A learner’s ability to acquire new information, therefore, begins by laying a strong foundation of knowledge and building robust schemas, and there are two ways to do this:
1 – Recalling Knowledge
An essential part of building schema is knowledge retrieval. When learners practice recalling information, they strengthen the connections between related pieces of information in their minds, making it easier to retrieve and use in the future. Regular and continued practice enables learners to lay a strong foundation of knowledge that supports the acquisition of new information
2 – Embedding Knowledge
Embedding information is the next crucial step; this involves connecting or linking new information to existing knowledge and experiences so that learners can achieve a deeper and more comprehensive understanding of the topic. At this stage, the learner can add further knowledge and links between concepts, contributing to a more robust schema and facilitating the development of higher-order thinking skills. However, for this to be effective, the learner must have established and be able to maintain a strong foundational knowledge base.
This image illustrates why this is critical to the learning process:
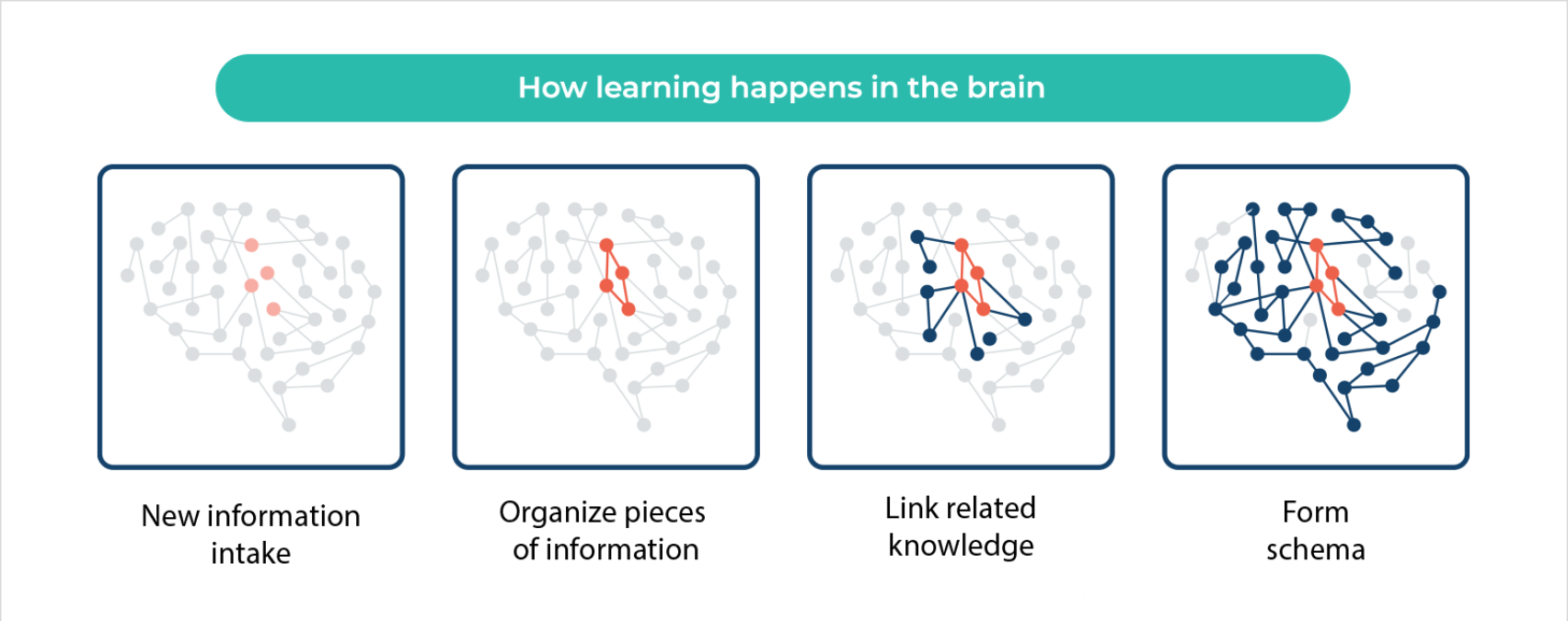
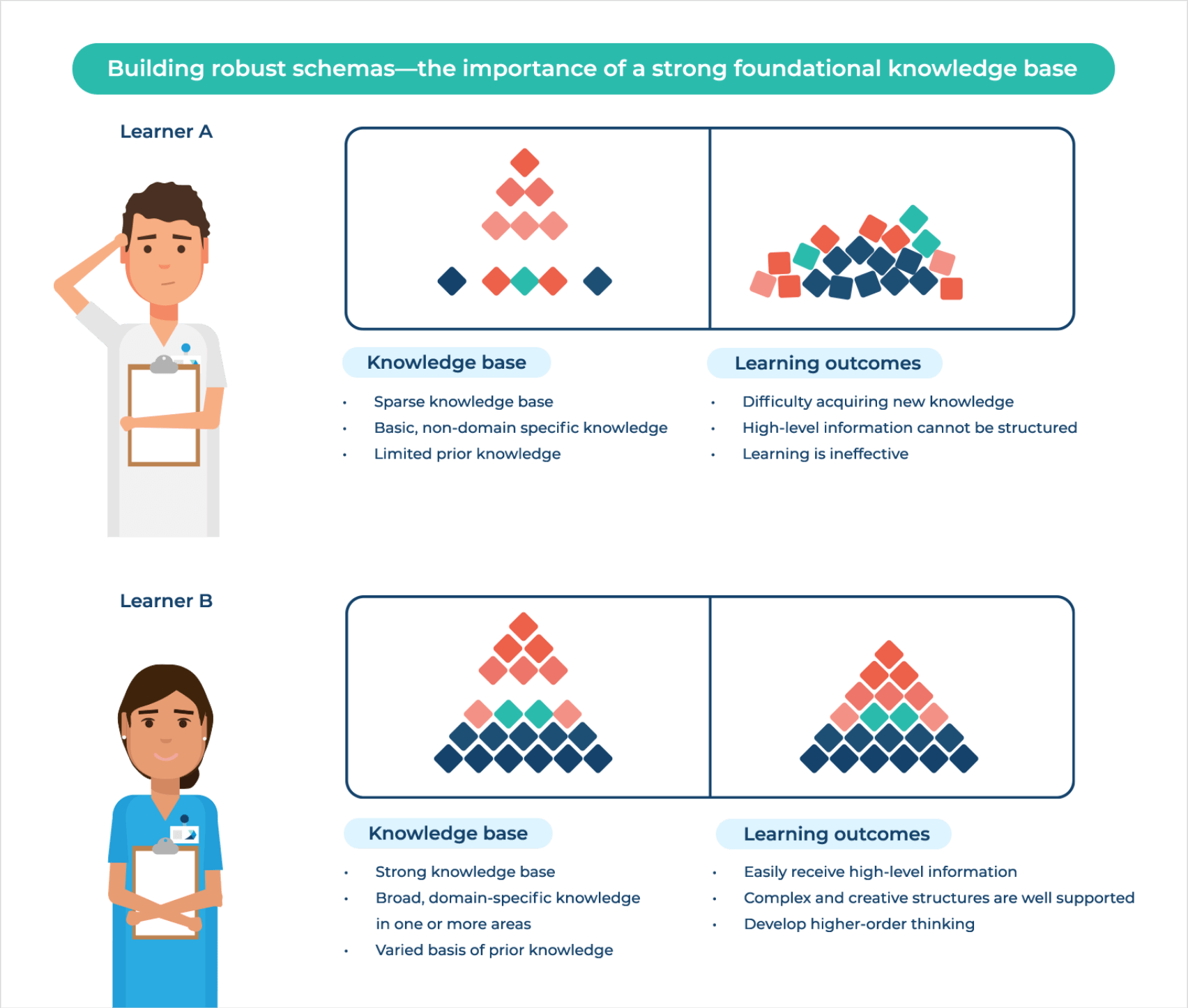
Higher-order learning abilities like critical thinking, clinical judgment, and creativity are dependent on the existence of broad and well-established domain-specific knowledge in one or more areas. Without this base, new high-level information cannot be structured appropriately and will not be useful. The wider and more varied the basis of prior knowledge, the higher, more complex, and creative structures it can support. Educational gaps and failures—sometimes, even attrition—arise when learners lack strong foundational knowledge and they struggle to build schema. It is therefore essential to help learners establish a solid knowledge base that can enhance their acquisition of new knowledge and develop higher-order thinking.
Cognitive load theory presents a powerful framework that empowers educators to drive this goal: by understanding how learning happens, educators can tailor their approach and instructional practices to optimize teaching in the classroom, resulting in more effective and efficient learning. They can draw upon the theory’s eleven principles or cognitive load effects to improve the quality of teaching and elevate learning outcomes.
11 Cognitive Load Effects That Help Elevate Learning
Cognitive load theory outlines these eleven effects that can be used to guide educators to reflect on their own practice and improve content delivery, leading to ideal learning performance and results:
Effects | Educators’ Takeaway |
---|---|
Element Interactivity9 | The higher the element interactivity level (related elements that need to be processed simultaneously in the working memory), the bigger the cognitive load. Reduce the load by adjusting the interactivity level to match learner’s expertise—consider the type and complexity of the information, and learner’s prior knowledge. |
Isolated Interacting Elements10 | Complex content with high element interactivity is best broken down and processed in isolation or it could be too much for the working memory to handle. The different chunks of information are stored in the long-term memory and later combined with more elements to help the learner achieve full understanding. |
Worked Example11 | Demonstrate the solution step-by-step to guide learners and help them understand the material more easily. This is known as “worked examples” and it works well for novice learners. When learners have mastered it, they can emulate the solution pattern to solve similar problems. |
Completion12 | Completion tasks are one level up from worked examples, in which learners—who are more advanced but still need some help and guidance—are provided with only partially completed examples. This still helps reduce their cognitive load but at the same time, leaves room for them to learn by filling in the gaps. |
Expertise Reversal13 | While novice learners generally benefit from instructional techniques that provide more guidance, the opposite could be true for advanced learners who have prior related knowledge—reduced guidance often results in better learning performance. |
Guidance Fading14 | As learners build up expertise and autonomy in learning tasks, instructional methods offering guidance and assistance should be decreased. More advanced learners should be allowed to solve problems independently. |
Redundancy15,16 | When covering new material, eliminating unnecessary information from lesson plans and avoiding presenting the same information in different forms—for example, reading text verbatim from a presentation during a lecture—helps decrease cognitive load and ensures that learners can focus on the relevant material that they actually need to learn. |
Split Attention17 | Content that’s presented as a graphic with text information provided separately requires learners to divide their attention between the two sources of information, reducing their capacity to learn. Overcome this by integrating both graphic and text into an infographic to focus the learner’s attention on a single source of instructional information, or leverage both visual and auditory channels to reduce cognitive load on the working memory, thereby minimizing the split attention effect.18 |
Goal-Free19 | When learners are asked to solve problems with a non-specific goal (“determine differential diagnosis”) rather than a clear objective (“identify root cause”), it lightens their cognitive load and encourages them to focus on the information provided to work backwards from the goal using a means-ends strategy, thereby improving learning. |
Modality20 | Visual and auditory cues are processed separately in the brain whereas similar cues will compete for space in the working memory. For example, a visual accompanied by audio narration (such as an audio-visual mnemonic) has a lower cognitive load compared to an image with written explanation (both visual). Pairing different information cues therefore helps minimize risk of cognitive overloading while optimizing the working memory’s capacity. |
Imagination20 | For the more advanced learner with a broader base of schemas, being asked to imagine the concepts and procedures may actually help them learn better than conventional studying or when presented with worked examples. This is because imagining helps improve the degree of automated association of schemas, enhancing learning performance. |
Understanding cognitive load theory and applying its principles to lesson planning and curriculum development enable educators to design effective strategies that help learners learn better and retain information longer. Learners will be empowered to build a solid knowledge base that’s primed for information acquisition and retention, paving their way toward mastery of essential content and complex concepts while cultivating lifelong learning habits that will shape their future practice and success.
Discover How Learning Science Can Be Leveraged to Optimize Knowledge Acquisition and Retention Among Healthcare Learners
References
1 Cowan N. Working memory underpins cognitive development, learning, and education. Educ Psychol Rev. 2014;26(2):197-223. doi:10.1007/s10648-013-9246-y
2 Cowan N. George Miller’s magical number of immediate memory in retrospect: Observations on the faltering progression of science. Psychol Rev. 2015;122(3):536-541. doi:10.1037/a0039035
3 Peterson LR, Peterson MJ. Short-term retention of individual verbal items. J Exp Psychol. 1959;58(3):193-198. doi:10.1037/h0049234
4 Researchgate.net. Accessed June 27, 2023. https://www.researchgate.net/publication/247130036_Perception_and_memory_in_chess_Heuristics_of_the_professional_eye
5 MindTools. (n.d.). Mindtools.com. Retrieved June 6, 2023, from https://www.mindtools.com/aqxwcpa/cognitive-load-theory
6 Cowan, N. (2001). The magical number 4 in short-term memory: A reconsideration of mental storage capacity. Behavioral and Brain Sciences, 24(1), 87-114. https://doi.org/10.1017/S0140525X01003922
7 InnerDrive. (2019, February 28). Using cognitive load theory in the classroom. Innerdrive.co.uk. https://blog.innerdrive.co.uk/using-cognitive-load-theory-in-the-classroom
8 Young, J. Q., Van Merrienboer, J., Durning, S., & Ten Cate, O. (2014). Cognitive Load Theory: implications for medical education: AMEE Guide No. 86. Medical Teacher, 36(5), 371–384. https://doi.org/10.3109/0142159X.2014.889290
9 Chen O, Kalyuga S, Sweller J. The worked example effect, the generation effect, and element interactivity. J Educ Psychol. 2015;107(3):689-704. doi:10.1037/edu0000018
10 Pollock E, Chandler P, Sweller J. Assimilating complex information. Learn Instr. 2002;12(1):61-86. doi:10.1016/s0959-4752(01)00016-0
11 Cooper G, Sweller J. Effects of schema acquisition and rule automation on mathematical problem-solving transfer. J Educ Psychol. 1987;79(4):347-362. doi:10.1037/0022-0663.79.4.347
12 Van Merrienboer JJG, Krammer HPM. Instructional strategies and tactics for the design of introductory computer programming courses in high school. Instr Sci. 1987;16(3):251-285. doi:10.1007/bf00120253
13 Sweller J, Ayres PL, Kalyuga S, Chandler P. The expertise reversal effect. Published online 2003:23. doi:10.1207/S15326985EP3801_4
14 Atkinson RK, Renkl A, Merrill MM. Transitioning from studying examples to solving problems: Effects of self-explanation prompts and fading worked-out steps. J Educ Psychol. 2003;95(4):774-783. doi:10.1037/0022-0663.95.4.774
15 Chandler P, Sweller J. Cognitive load theory and the format of instruction. Cogn Instr. 1991;8(4):293-332. doi:10.1207/s1532690xci0804_2
16 Kalyuga S, Chandler P, Sweller J. Managing split-attention and redundancy in multimedia instruction. Appl Cogn Psychol. 1999;13(4):351-371. doi:10.1002/(sici)1099-0720(199908)13:4<351::aid-acp589>3.0.co;2-6
17 Tarmizi RA, Sweller J. Guidance during mathematical problem solving. J Educ Psychol. 1988;80(4):424-436. doi:10.1037/0022-0663.80.4.424
18 Mayer RE, Moreno R. A split-attention effect in multimedia learning: Evidence for dual processing systems in working memory. J Educ Psychol. 1998;90(2):312-320. doi:10.1037/0022-0663.90.2.312
19 Larkin J, McDermott J, Simon DP, Simon HA. Expert and novice performance in solving physics problems. Science. 1980;208(4450):1335-1342. doi:10.1126/science.208.4450.1335
20 Mayer AR, Franco AR, Canive J, Harrington DL. The effects of stimulus modality and frequency of stimulus presentation on cross-modal distraction. Cereb Cortex. 2009;19(5):993-1007. doi:10.1093/cercor/bhn148